Pathophysiology of Chronic Thromboembolic Pulmonary Hypertension and Chronic Thromboembolic Disease
Chronic thromboembolic disease (CTED) is believed to develop when acute pulmonary emboli (PE) fail to dissolve by autolysis after a 3-month period of anticoagulation. Residual defects can be identified in up to 50% of patients when studied using lung perfusion scintigraphy 3–12 months after an acute PE.1,2 The thromboembolic material is replaced by fibrotic material, develops an endothelial covering and cannot be cleared by anticoagulation or thrombolytic therapy.3 Despite the prevalence of residual intravascular material, the development of pulmonary hypertension is uncommon – reported to be <1% in clinical practice, and 2–3% in the prospectively followed Pulmonary Embolism Thrombolysis (PEITHO) study.4,5
Chronic thromboembolic pulmonary hypertension (CTEPH) is present when, in addition to CTED, pulmonary pressures are raised to abnormal levels and is usually associated with significant effort limitation. Since the 6th World Symposium on Pulmonary Hypertension (WSPH), this has been redefined as a mean pulmonary artery pressure (mPAP) of >20 mmHg with a pulmonary vascular resistance (PVR) of ≥3WU.6 However, prior to 2019 the convention was to diagnose pulmonary hypertension only where the mPAP was ≥25 mmHg – thus all studies published to this date relied on the previous definition. This is an important distinction to acknowledge when applying trial data to clinical practice.
Pulmonary hypertension in CTEPH does not necessarily correlate with the volume of obstructive material, but is augmented by an additional vasculopathy that develops months to years after the initial event.3,4 Among those who develop CTEPH, pulmonary hypertension usually fails to resolve after an acute PE with breathlessness persisting and worsening over time as the associated vasculopathy progresses. In 23% of cases there is no obvious acute preceding event.7 Whether this implies a subclinical initial event followed by progressive vasculopathy is not yet established.
Management Options in Chronic Thromboembolic Pulmonary Hypertension
The established intervention for managing CTEPH is surgical pulmonary endarterectomy (PEA).4 This is technically feasible in 60% of patients with CTEPH and is associated with excellent symptomatic, haemodynamic and prognostic outcomes.7 In-hospital mortality has been reported at around 5% in the international CTEPH registry, although the largest centres currently report a 30-day mortality of 2.2%.7,8 Three-year survival has been reported at 89% in operated patients versus 70% in unoperated patients.7 In patients deemed surgically operable but who declined intervention, only 55% survived to 5 years compared to 83% who underwent PEA.9
In patients with inoperable or post-PEA CTEPH, medical therapy with riociguat has been shown to improve 6-minute walk distance and modestly reduce mPAP (<10%) and is the only licensed therapy for this condition.10 Long-term data suggesting prognostic benefit are based on open-label follow-up in the BAY63-2521 – Long-term Extension Study in Patients With Chronic Thromboembolic Pulmonary Hypertension (CHEST-2) trial with an estimated 93% 2-year survival rate among the 62% of the study population who continued therapy for at least 2 years.11 Unlicensed medical therapy (phosphodiesterase inhibitors and endothelin receptor antagonists) was not associated with improved survival in the international CTEPH registry.7
The first series of balloon pulmonary angioplasty (BPA) was reported nearly 20 years ago by Feinstein et al.12 The technique has subsequently been refined in multiple centres in Japan and is now rapidly being adopted in Europe and the US.13–19
Balloon Pulmonary Angioplasty
Development
The first report of BPA for CTEPH was by Voorburg et al. in 1988, who dilated four lesions in a 30-year-old patient, reducing mPAP by 24% (from 46 to 35 mmHg).20 Parallel work in animal models and patients with congenital stenoses provided insights into the required degree of stenosis dilation and maximum tolerated balloon diameter to vessel ratio.21 Given this background, improvements in angioplasty equipment and the poor outcomes in patients who were not candidates for PEA surgery, Feinstein et al. performed BPA in 18 patients deemed either to have non-surgical disease (n=16) or being unfit for surgery (n=2).12 Patients underwent an average of 2.7 BPA sessions, targeting an average of six lesions per patient. Mean PAP fell by 9 mmHg without a change in cardiac index, symptoms (New York Heart Association score improved by 1.5) and walk distance improved by 288 m. However, adverse events were common. One patient died 7 days post procedure and 11 developed reperfusion oedema, three requiring mechanical ventilation.
In Europe and the US, the high complication rate associated with BPA led to a focus on refining PEA and developing medical therapies for CTEPH. However in Japan, work on refining BPA continued. A decade later, a number of publications from several centres demonstrated that BPA performed in a staged fashion reduced the risk of lung injury, was associated with significant haemodynamic and symptomatic benefit and appeared to improve survival.13 By 2013, as progress with medical interventions was proving slow and approximately one-third of patients were still deemed to have surgical inaccessible disease, major European centres began to perform BPA. Table 1 outlines the registries published to date (excluding, where possible, those covering the same population more than once).22–36
Imaging Modalities
Selective pulmonary angiography is an essential as part of the BPA procedure. However, selecting patients and target territories requires familiarity with several other modalities. Most centres use a multimodal assessment, including lung scintigraphy, CT pulmonary angiography and high-quality invasive pulmonary angiography, to identify targets, with the latter providing information on the dynamic flow through target lesions, localised parenchymal perfusion and venous return.19,30 While useful for proximal disease, MR pulmonary angiography currently has a limited role in imaging the segmental and often subsegmental disease targeted in BPA, although cardiac MR to document the right ventricle (RV) state before and in response to BPA is clearly of value.37
Dual energy CT scanning has the potential to combine the strengths of scintigraphy and CT scanning, providing anatomical and perfusion imaging with a single investigation.38 However, this is an expensive modality with limited general applicability, and so single energy subtraction CT is being explored because it offers similar information without the need for dedicated equipment.39 C-arm CT 3D subtracted imaging (cone beam CT) of the pulmonary arteries has also been reported.22,32 The superior soft tissue imaging allows tracing of the course of occluded vessels and blending with fluoroscopic images allows tracing of the direction for guidewire advancement in three dimensions. In addition, superior resolution allows detail of the lesions to be resolved prior to intervention (webs, slits, subtotal and total occlusions) and allows accurate planning of required balloon size. While laborious, this has been associated with very low complication rates (no complications in 89% of 266 sessions and only 1.5% experiencing complications that required active management).32 Similar information is available non-invasively with a more readily available technique of ECG gated 320-row area detector CT scanning.22
Intravascular ultrasound (IVUS) has been used to accurately size vessels to be treated thus avoiding over-inflation and vascular damage, with virtual histology being explored to identify lesions most likely to respond to balloon dilation.40,41 Optical coherence tomography (OCT) provides unparalleled detail of the lesions being treated but at the cost of substantially greater contrast administration.42 From OCT studies we have learned that the standard ambition of dilating lesions to 0.8 of the reference vessel size is not currently achievable in most (70%) of lesions because of the complex nature of the lesions being treated (thick multi-hole webs) or elastic recoil (thick walled mono-hole).42
Procedural Details
BPA is carried out via either the femoral or jugular route and guiding catheters passed to the pulmonary arteries. Buffeting of the catheter by the right ventricle and movement of the pulmonary vasculature with respiration is minimised by the use of a long (65–80 cm) sheath and instrumenting target vessels during breath-hold.18,34 Most operators have found that hydrophilic guidewires facilitate crossing of complex webs, but these need careful handling to minimise the most common complication – wire perforation (Table 1). Target vessels are segmental and subsegmental vessels, although lobar vessels (more usually a target for PEA) have been treated.22 The most common balloon sizes are 2–4 mm, although larger sizes (up to 14 mm) have been reported.16–18,34,43,44 The aim is to restore normal flow with rapid filling of the draining pulmonary veins, although pressure wire and microcatheters have been used to achieve a distal pressure of 0.75–0.8 of proximal pressure where mPAP is <35 mmHg.43,44
Targets for treatment depend on lesion characteristics (webs, stenoses and tapered occlusions/subtotal occlusions in preference to abrupt occlusions; Figures 1–3) in areas of reduced parenchymal perfusion.19 The success rate is highest (99%) and complication rate lowest (2%) for ring and web lesions, while the success rate is lowest for total occlusions (52%) and the complication rate highest in tortuous vessels (43%).45 In territories where perfusion is reduced but no lesion is visible at angiography, one may still encounter webs that require dilatation; thus perfusion defects are regarded as more important than angiographically visible lesions in choosing segments to interrogate.19 Because of the greater physiological impact, lower lobe vessels are prioritised and the right lung over the left lung. Guidewire support using a balloon or microcatheter is not unusual, but more aggressive techniques such as wire escalation is avoided where possible as the risk of wire exit increases significantly.18 Standard semi-compliant balloons are used for most procedures and cutting balloons are generally avoided – again to reduce complications in these thin-walled mobile vessels.19
Most patients require multiple BPA sessions (three to five being typical) and the interval between sessions varies from 3 days to 1 month or more (Table 2).18,19,46,47 Individual procedures are limited by the volume of lung treated (usually one or two lobes, although more extensive has been described), radiation dose (<2 Gy) and contrast volume (<4–6 ml/kg).19 Stenting is unnecessary as lesion recoil/recurrence is rare (three of 1,897 lesions restudied after >1 year had occluded).25 The exception is covered stents for major vascular injury.
Efficacy
A supportive evidence base is emerging for efficacy, including a meta-analysis suggesting superiority of BPA when compared to riociguat.48 The recently completed randomised controlled Riociguat Versus Balloon Pulmonary Angioplasty in Non-operable Chronic Thrombombolic Pulmonary Hypertension (RACE) trial (ERS 2019 abstract), included 124 patients randomised 1:1 to either BPA or riociguat. After 6 months PVR fell by 60% in the BPA group and 32% in the medical therapy group (p<0.001).49 The secondary endpoints change in mPAP, mean right atrial pressure, N-terminal pro-brain natriuretic peptide (NT-proBNP) and functional class (FC) showed greater improvement in the BPA group, although 6-minute walking distance (6MWD) was not significantly different between the two groups. Unsurprisingly, adverse events were significantly more common following BPA (50% versus 26%), although severe adverse events were less common (14% versus 9%) with haemoptysis and lung injury dominating in the BPA group and gastrointestinal adverse effects in the riociguat group. However, given their different sites of action, medical therapy and BPA are regarded as complementary.50
Registry data also support the role for BPA confirming a substantial reduction in mPAP, PVR and increase in cardiac index in patients undergoing BPA (Table 1). The haemodynamic effects are not seen during the procedure, but rather documented at follow-up at 52 ± 81 days.51 The actual timing of the benefit is unclear; however, among the 14 patients studied by Hosowaka et al, five were restudied within 1 month and pressures were already reduced.51 In another study, no further reductions were evident between 1 week after the final procedure and follow-up 2 years later.52
FC is also consistently improved, with the vast majority of patients achieving FC I/II after BPA. The 6MWD improved by 50–100 m in almost all studies (Table 2). Most studies have included measurement of brain natriuretic peptides and all have reported substantial reductions in these markers of myocyte stretch.
As expected from PEA data, improvement but not normalisation of right ventricular function post BPA is consistently documented.18,24,53,54
Although the evidence for mortality benefit is weak to date, there is some available. Siennicka et al. reported 58 patients undergoing BPA compared to 67 treated with medical therapy.31 Survival was superior in the BPA population at 92% (95% CI [85–99%]) versus 79% (95% CI [69–90%]) in those randomised to medical therapy during 22 months of follow-up. In addition, Tanigushi et al. reported improved survival in the French cohort since the introduction of BPA and with current practice BPA, but not medical therapy, was an independent predictor of survival (HR 0.297; CI 95% [0.098–0.907]).55
The BPA treatment effect appears to be durable. Inami et al. reported that among 30 of 57 eligible patients who underwent follow-up catheterisation after 50.3 months (interquartile range 47.4–55.6 months), mPAP and PVR remained >50% lower than baseline values.56 Ogawa et al. identified 196 patients who had undergone follow-up catheterisation at 425 ± 281 days after the last procedure.25 The authors reported near normal pressures and low rates of medical therapy usage (mPAP 22.5 ± 5.4 mmHg; PVR 288 ± 195 d.s.cm−5, 45% on medical therapy compared to 70% prior to BPA).
Safety
At the recent WSPH complications of BPA have been classified as shown in Table 3.6 Lung injury is the main issue; while this was previously thought to be due to reperfusion oedema, more recent data suggest that vascular trauma is the more likely explanation. Table 4 summarises the studies reporting adverse events in sufficient detail. In general, minor complications occur in 10–15% of sessions, but the largest study reported adverse events in 36%.25 The long-term significance of minor adverse events is yet to be determined although serious adverse events appear to be uncommon (Figures 4 and 5).
Higher rates of lung complications are reported when using routine chest CT scanning after every procedure. Ikeda et al. reported on 30 patients whp underwent BPA to 879 lesions in 112 BPA sessions.34 Pulmonary artery perforations were identified in 21 lesions at angiography, but 93 hyperdense lesions (compatible with parenchymal haemorrhage) were observed at CT performed within 15 minutes of completion of BPA. Analysing by lesion showed that around 10% of web, ring and abrupt narrowing were associated with haemorrhage, while nearly 40% of occlusive lesions treated were associated with haemorrhage. Ejiri et al. confirmed these findings in a further CT study of 76 patients undergoing BPA to 1,247 vessels.57 In this study, vessel injuries identified at angiography were the strongest predictor of lung injury identified on CT scanning performed within 24 hours of the procedure (33% versus 2.5%). Of note, there is a clear learning curve, with experienced centres observing a significant reduction in adverse event rates in more recently treated patients (complication rates falling from 11.2% in the first 1,006 sessions to 7.7% in the most recent 562 sessions).30 Newer centres are learning to avoid the initial high complication rates, with Godinas et al. reporting a 10% complication rate in the first 90 procedures, only one of which was severe.35 Peri-procedural mortality is generally reported to be approximately 2% per patient and is similar to PEA surgery in the best centres.58

Outstanding Issues
Several remaining issues need to be resolved to improve the efficacy and safety of BPA.
Pathophysiology of Chronic Thromboembolic Pulmonary Hypertension
From limited intravascular imaging and histology, we have a general sense of the nature of the lesions being treated and the mechanism of benefit.42,59 The lesions being addressed are largely fibrotic webs and stenosis, and much of the increase in cross sectional area is achieved through expansion of the vessel rather than compression of the intraluminal material.42,57,60,61 In a proportion, haemodynamics fail to improve despite clearing all the proximal disease.62 Whether this is due to persistent microvascular disease or distal unseen lesions that might be a target for intervention is, as yet, unresolved.63
The precise mechanism of benefit requires further evaluation. There is no immediate effect on pulmonary pressures – that appears later.51,52 Yet simultaneous haemodynamic and IVUS interrogation shows that pulmonary artery compliance increases immediately with no impact on distensibility or compliance at the site of treatment.59 In addition, further enlargement of the vessel tends to occur in the weeks to months post BPA, presumably induced by flow-mediated dilatation.44 More detailed understanding of this process will clearly be important going forward, to enable titration of treatment to effect in real time.

Advances in Imaging Supporting Balloon Pulmonary Angioplasty
As mentioned above, several novel imaging techniques have the potential to improve outcomes in BPA. Subtraction CT by imaging diseased vessels supplying co-localised hypoperfused areas, could facilitate differentiation between disease amenable to intervention and microvascular disease. ECG gated area CT or cone beamed CT may identify treatable disease inadequately resolved on pulmonary angiography. Developments in MR perfusion mapping may create a role for MR in selecting territories to target and in monitoring changes in lung perfusion in response to BPA, reducing total radiation dose associated with repeated scintigraphy or CT scanning. Further work is necessary to define which of these techniques add value and should be incorporated into routine clinical usage.
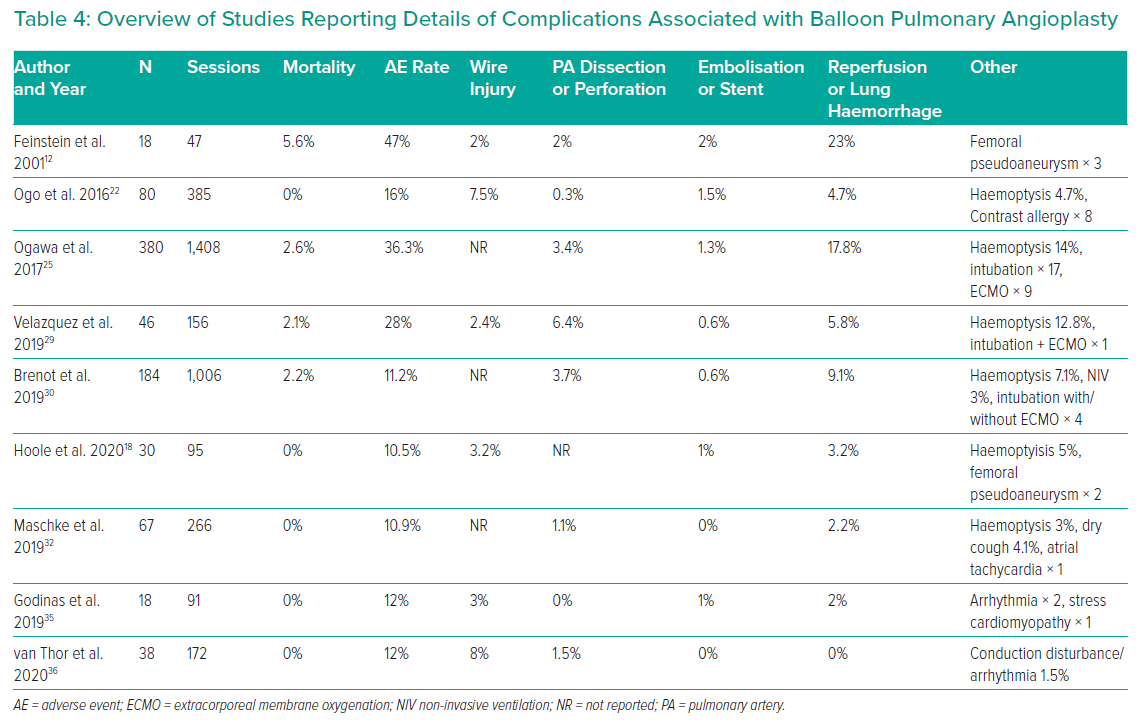
Virtual histology on IVUS or OCT add considerable complexity to the BPA procedure, but may provide insights into the optimal lesions to target, such information combined with improved non-invasive imaging may help further refine target lesion selection.
The Optimal Interventional Approach
To achieve optimal results safely, two main approaches are currently practiced. Addressing all accessible segments in each lung initially using a small balloon (2 mm), returning to that lung after a week or more with optimally sized balloons.22 The downside of this strategy is wiring large numbers of vessels at each session – wire trauma is thought to be the most common cause of lung injury.34,55 The alternate approach is limiting treatment the number of segments treated until the mean PAP is below 35 mmHg when a more aggressive approach is tolerated. We need to determine which of these approaches is optimal.18
Dedicated equipment for BPA has not been a focus to date. Our industry partners are unlikely to invest resources unless the numbers of procedures performed increase significantly. Guide catheters, guide wires, intravascular imaging devices, etc., have all been borrowed from the coronary field and are not optimised for use in the larger, more mobile and thin-walled pulmonary vasculature.
Given the data that complications are more severe among patients with very high pulmonary pressures, there is a logic to optimising medical therapy prior to BPA.42 Studies are required to determine whether optimising haemodynamics before BPA reduces the risks of the procedure.64
Novel Populations: Who Should be Offered Balloon Pulmonary Angioplasty?
The evidence base at present supports PEA surgery for proximal disease. However, registry data from Japan are beginning to suggest that BPA may eventually be in a position to challenge this orthodoxy (Table 1). A large international registry currently underway (NCT02656238) should provide evidence to allow planning of a BPA versus PEA trial in the next few years. The granularity of data in this registry will hopefully provide insights as to whether there are subgroups (such as those with dominantly occlusive disease) that should not be randomised and whether equivalent haemodynamic and symptomatic outcomes can be achieved.
A second possible role of BPA is to lower the risk of intervention in patients where there are very adverse haemodynamic profiles or co-morbidities that render surgical risks unacceptable.65,66 If we can determine from the registry that in very high-risk patients BPA is associated with lower risks than PEA and that BPA does not disrupt the dissection plane for the surgeon, then BPA may well facilitate lower risk PEA in those with adverse haemodynamics. In the frail population we will need to confirm that BPA still confers net benefit.67
It is evident from published registries that BPA is both safe and effective in at least a proportion of the post-PEA population.25,31 However, this may depend on either inadequate initial clearance or recurrent disease. Where distal vasculopathy is the dominant driver for residual pulmonary hypertension, medical therapy may be the only effective option.56 In addition, not all lesions are surgically accessible in any individual patient so a hybrid approach with the surgeon focusing on proximal lesions and BPA to clear distal disease has been reported.68 Further work is required to fully characterise the population undergoing PEA and to understand where the risk benefit ratio favours a role for BPA either as part of a hybrid approach or for residual pulmonary hypertension post PEA.
Because of the low risk of complications associated with BPA, particularly in patients with near normal haemodynamics, some centres have performed BPA on patients with CTED without pulmonary hypertension.69,70 Proving that the risk–benefit ratio is favourable in this population is fraught with difficulty – only with a sham control group can superiority be established, given the substantial placebo effect of intervention.71
Agreeing the Goals of Treatment
The ultimate goal of any intervention must be to improve symptoms, effort tolerance and prognosis. The goals must be tailored to the individual. Elderly patients may be satisfied with modest improvements in effort tolerance, in young patients normalisation of prognosis and exercise capacity are important objectives, while in patients with co-morbidities there will be limits to that which can be achieved by addressing the pulmonary vasculature and a different risk benefit balance. The procedural objectives, which are surrogates for these objectives, have yet to be validated. The initial approach was simply to tackle all accessible lesions if the mPAP remained >30mmHg.9 Variations are now emerging with some recommending a limited approach of predominantly targeting lower lobe vasculature (where the benefit is maximal and the risk lower).18 Most Japanese centres set a haemodynamic endpoint – typically an mPAP of <25 or even lower.22,25 Mahmud et al. suggest targeting improved perfusion on scintigraphy.19 Which of these approaches will yield the optimal risk–benefit ratio remains to be determined.
Another possible approach would be to target right ventricular recovery, since it is right ventricular failure that determines prognosis. Whether through simple assessments of RV size and systolic function or more logically aiming to normalise RV-PA coupling or energetics, remains unclear.18,24,27
Initial studies from Japan have reported excellent haemodynamic outcomes while reducing adjuvant drug usage, following the example set in PEA surgery.25,58 Yet it is clear that CTEPH is a complex disease process where patients have a vasculopathy as well as obstructive disease.3 It is reasonable to suggest that on-going medical therapy may have a role post BPA to ensure optimal durability of the clinical benefit, but this has yet to be investigated in controlled studies.
Conclusion
BPA is now an established treatment modality for patients with inoperable CTEPH that – like coronary angioplasty – is destined to become part of standard therapy for this condition. We need to learn from the experience in coronary angioplasty and ensure that we do not allow BPA practice to expand without an adequate evidence base. Adequately powered randomised controlled trials and strong guideline-based limitations on practice are essential to achieve the best BPA results and improve outcomes of patients with CTEPH.