End-stage heart failure (HF) accounts for a large proportion of deaths in developed countries and the gold standard treatment is orthotopic heart transplantation. Due to the growing population of patients with advanced HF and the ongoing shortage of available organs, mechanical circulatory support (MCS) with long-term left ventricular assist devices (LVADs) has become a common treatment for patients with severe left ventricular systolic dysfunction who no longer respond to optimal medical therapy (OMT). LVAD therapy improves outcomes in HF patients by unloading the left ventricle (LV), increasing cardiac output and lowering intracardiac pressures. As a result, LVADs enhance peripheral circulation and maintain end-organ perfusion, improve functional capacity and relieve HF symptoms.1
MCS has been historically used to ensure the survival of patients with end-stage HF until a donor organ becomes available, a strategy referred as a bridge to transplantation (BTT). Several studies have demonstrated that LVADs when used as a BTT reduce mortality by improving the patient´s overall condition before heart transplantation and also improving post-transplant survival.2–5 Furthermore, BTT strategy represents an especially effective alternative for patients with advanced HF who are young, have renal dysfunction or expect prolonged waiting times.6 LVAD implantation as a BTT also improves the quality of life of patients with end-stage HF by allowing them to leave hospital while waiting for heart transplantation.
A growing number of patients in some countries have received LVADs as a permanent treatment or ‘destination therapy’ (DT) since the publication of the REMATCH trial in 2001. REMATCH demonstrated improved survival in patients with advanced HF who are ineligible for heart transplantation who were treated with LVADs versus OMT. Another group of patients who may potentially benefit are those with comorbidities that represent relative contraindications to heart transplantation and may be reversible after a period of mechanical haemodynamic support; a strategy known as a bridge to decision (BTD).7 Furthermore, a very small proportion of LVAD patients have underlying causes of systolic dysfunction that may be reversible over time and they may recover sufficient cardiac function to allow withdrawal of MCS.8 According to the Interagency Registry for Mechanically Assisted Circulatory Support (INTERMACS) database, between 2008 and 2017, 26% of patients with LVADs were listed for cardiac transplantation at the time of LVAD implant (BTT), 43% as DT, 30% as BTD and less than 1% of LVADs were explanted after sufficient recovery of LV systolic function.9
Most patients treated with implantable LVADs receive an intracorporeal rotary pump that unloads the failing LV continuously by pumping blood to the ascending aorta, either an axial flow pump such as the HeartMate II (HM2; Abbott), a centrifugal pump such as the HeartWare HVAD (Medtronic) or the new generation magnetically levitated centrifugal pump HeartMate III (HM3; Abbott). In June 2021, Medtronic stopped distribution and sale of the HeartWare HVAD, meaning that the HM3 device is currently the sole durable LVAD available for new implants.
Regardless of their design, all have the components shown in Figure 1: an inflow cannula surgically implanted into the LV apex conducting blood from the LV to the pump, which houses an impeller that impulses the blood into the systemic circulation through an outflow graft inserted in the aorta; a dual set of external batteries that provide power to an external controller that operates and monitors the pump function; and a surgically tunnelled driveline that connects the pump to the system controller. The pumps generate up to 10 l/min of flow, which is calculated based on measured pump power and set pump speed.10
Right heart catheterisation (RHC) is a key investigation in the assessment of patients being considered for LVAD implantation and heart transplantation. Haemodynamic evaluation using RHC remains the gold standard to providing objective metrics of right ventricle (RV) function, pulmonary pressures and cardiac output. This review outlines the use of RHC prior to LVAD implantation for prognostic assessment in the perioperative period, when evaluating postoperative complications, and when assessing for bridge to recovery.
Overview of Right Heart Catheterisation
RHC plays a significant role in the prognostic evaluation and decision-making for patients with end-stage HF. The route of access depends on several factors, including operator expertise, the presence of cardiac devices, previous history of venous cannulation and associated complications.11 Femoral vein access is commonly used if left heart catheterisation is jointly performed, although some studies have demonstrated the safety of performing RHC and left heart catheterisation via the antecubital fossa vein and radial artery.12–14 A meta-analysis comparing landmark-based versus ultrasound-guided venous access demonstrated a clear benefit of ultrasound for internal jugular vein (IJV) cannulation, with a higher success rate and fewer complications.15 RHC can be performed without interrupting anticoagulation in patients undergoing RHC via either IJV or antecubital veins with an international normalised ratio (INR) <3.5.16
Complications associated with RHC are uncommon in modern clinical practice; however, when they do occur the consequences can be severe and sometimes fatal. Case reports or case series are the main source of reported complications, and they can be categorised as related to either vascular access or the catheterisation procedure itself. The most frequent injuries related to access are carotid artery injury and the formation of arteriovenous fistula. Other access site-related complications include pseudoaneurysm of the common femoral vein, perforation of the right innominate vein, deep venous thrombosis, perforation of the left internal mammary and right lymphatic duct injury. Access-site complications can be significantly reduced by performing ultrasound-guided vessel puncture. The most reported catheter-related complication is injury to the tricuspid valve. Pulmonary artery (PA) rupture carries a high mortality rate in the reported literature; however, it occurs in less than 0.5% of cases. Other catheter-related complications include catheter knotting or bending, right atrial (RA) perforation and severe cardiac arrythmias.17
Right Heart Catheterisation Prior to LVAD Implantation
RHC in a patient being evaluated for advanced HF therapies should help to answer the following questions (Table 1):
- Do the cardiac haemodynamics indicate a poor prognosis, thus suggesting a potential benefit from transplantation or MCS?
- Are the pulmonary pressures low enough to enable safe transplantation?
- If LVAD therapy is being considered, what is the risk of post-implantation RV failure?
Haemodynamics as a Prognostic Indicator in End-stage Heart Failure
RHC remains an important test for assessing the need for MCS as well as maintaining candidacy for heart transplantation. As per the International Society for Heart and Lung Transplantation (ISHLT), RHC remains a class 1 recommendation for all candidates before being listed for heart transplantation and it should be performed annually until transplantation.18 Several studies have highlighted the role of clinical and haemodynamic profiling of patients with HF in predicting the risk of clinical deterioration and short-term survival.19,20 Four haemodynamic profiles have been characterised defined by the presence or absence of pulmonary venous congestion (pulmonary capillary wedge pressure [PCWP] >15 mmHg or PCWP <15 mmHg) and adequacy of perfusion (cardiac index >2.2 l/min/m2):
- Profile I: no congestion or hypoperfusion;
- Profile II: congestion without hypoperfusion;
- Profile III: hypoperfusion without congestion; and
- Profile IV: both congestion and hypoperfusion.
Several clinical features can accurately predict haemodynamic derangements in HF. Orthopnea and a New York Heart Association (NYHA) class III/IV correlate with elevated PCWP, renal dysfunction with elevated RA pressure, and hepatic dysfunction with increased PCWP and RA pressure.

Patients with pulmonary venous congestion, elevated RA pressure and low cardiac output have been identified as the group with the highest risk of death or clinical deterioration.21–23 Identification of this cohort of patients with poor prognostic markers is essential to evaluate the potential benefit of heart transplantation or LVAD therapy.
Pulmonary Pressures Assessment in Advanced Heart Failure
Progression of HF alters the pulmonary circulation as a consequence of chronically elevated left atrial and post-capillary pressures, leading to changes in the pulmonary vasculature that over time become irreversible. Pulmonary vasoconstriction and vascular remodeling ultimately lead to fixed pulmonary hypertension (PH). Elevated PA pressure and pulmonary vascular resistance (PVR) in the transplant recipient are associated with an increased risk of failure of the non-conditioned donor RV following transplantation, which leads to significant morbidity and mortality in the early post-operative period. ISHLT considers a PVR >5 Wood units and trans-pulmonary gradient (TPG) >15 mmHg as contraindications to transplantation.24–26 LVAD implantation in type 2 PH frequently leads to significant reductions in TPG and PVR and this is attributed to the LVAD´s ability to mechanically unload the LV and subsequently reduce left-sided filling pressures (Figure 2).27–31 Therefore, LVAD implantation offers an opportunity for patients with advanced HF and type 2 PH without other comorbidities to become eligible for heart transplantation.
Assessing the Risk of Right Ventricular Failure Post-LVAD Implantation
Due to the increasing patient population eligible for long-term mechanical support, RV failure post-LVAD implantation has become more commonly encountered in clinical practice. Therefore, assessment of RV haemodynamics is particularly important before LVAD placement.32 Given that LVADs only support the LV, RV function needs to be sufficient to overcome the PVR to adequately fill the LV and therefore maintain systemic circulation. RV function may be impaired due to a cardiomyopathic process or coronary artery disease and needs to be able to tolerate the increased preload stress from additional LVAD flows and also maintain contractility. Several predictors of post-LVAD RV failure have been identified but there is no consensus on which measures of RV function would constitute an absolute contraindication for LVAD implantation.33–36 As a result, accurate preoperative prediction of RV failure post-LVAD implant remains a significant challenge.
One of the main risk factors for post-operative RV failure is preoperative RV dysfunction. However, RV function can improve post-LVAD implantation as a result of decongestion of the LV, lowering filling pressures and reducing pulmonary pressures.37 Assessment of the risk of RV failure post-LVAD implantation should be evaluated not only through haemodynamic and echocardiographic variables, but also by taking into account additional clinical factors. The most consistent preoperative factors associated with the development of RV failure include the need for mechanical ventilation or renal replacement therapy.38 Additionally, patients with more severe HF, as measured by the INTERMACS score, are at greater risk of RV failure.39 LVAD device type does not appear to influence risk of RV failure, with similar rates seen between axial and centrifugal flow pumps in the MOMENTUM study.40
Specific echocardiographic predictors of RV dysfunction have exhibited poor reproducibility across studies and should not be taken solely into consideration when addressing LVAD implantation. Puwanant et al. reported that a tricuspid annular plane systolic excursion <7.5 mm provided a specificity of 0.9 and a sensitivity of 0.46 for prediction of RV failure, whereas Kukucka et al. reported that an RV-to-LV end-diastolic diameter ratio >0.72 by transoesophageal echocardiography showed a sensitivity of 0.80 and specificity of 0.74 for RV failure after LVAD placement.41,42
Haemodynamic parameters identified as potential RV failure predictors in single-centre studies include a low RV stroke work index (RVSWI) and a RA to pulmonary capillary wedge ratio (RA:PCWR) greater than 0.63.43,44 PA pulsatility index (PAPi) is the difference between the PA systolic and diastolic pressures, divided by the central venous pressure. A PAPi <1.85 was identified as the optimal cut-off to determine an increased risk of early RV failure.45 A more recent study by Gonzalez et al. assessed the use of serial testing, by combining an initial PAPi with one following optimisation with diuresis and inotropes.46 A low combined PAPi was independently associated with higher risk of early RV failure, highlighting the value of determining RV contractile reserve through dynamic assessment.

RV prediction scores have been developed combining multiple preoperative variables. One of the first algorithms was the Michigan RV failure risk score, which incorporated perioperative variables like vasopressor requirement, renal dysfunction and elevated bilirubin levels.47 A more contemporary risk score was developed by Kormos et al. using the Heartmate II BTT data, and found that a CVP:PCWP >0.63, a blood urea nitrogen greater than 39 mg/dl, or the need for preoperative ventilatory support were associated with RV failure.48 The EUROMACS score, which incorporates five clinical variables, includes one haemodynamic measure – RA:PCWP ratio.39 The c-statistic of 0.7 in the development cohort was better than other prediction tools, however it performed less well in small-to-moderately sized single-centre external validation cohorts.49,50 This has also been the case for validation of other risk prediction tools, which have demonstrated modest-to-poor discriminatory ability in external populations.
Haemodynamics Following LVAD Implantation
In general intensive care practice, the use of PA catheters has declined over recent decades. Nevertheless, in cardiac surgery PA catheters are still commonly placed to facilitate perioperative management. Following LVAD implantation, the continuous LV unloading produces a drastic reduction on PCWP and mean PA pressure and an increase in cardiac output. The reduction of RV afterload improves the RVSWI and tricuspid regurgitation. Furthermore, Goodwin et al. demonstrated that LVAD implantation frequently corrects functional mitral regurgitation without any concomitant perioperative mitral valve intervention.51 Comparison of haemodynamics among different devices is difficult as haemodynamics are also dependent on each centre’s perioperative management protocol and every patient’s previous condition.
Haemodynamic assessment in the early post-operative period can also facilitate the diagnosis of potential life-threatening complications, including acute RV failure and cardiac tamponade secondary to post-procedure pericardial bleeding or localised haematoma.
Acute Right Ventricular Failure
Acute RV failure in the early post-operative period may develop in patients after LVAD implantation. This is characterised by elevated central venous pressure (CVP), RA and PA pressures with reduced LVAD flows and cardiac output requiring RV support (inotropic or mechanical) for more than 14 days post-LVAD surgery. Severe RV failure after LVAD implantation is associated with an important increase in morbidity and mortality and less successful bridging to cardiac transplantation.33,48–50,52–54 The incidence of post-operative RV failure ranges from 5% to 44%, in part due to the varying definitions of RV failure in this patient population.55 Early perioperative RV failure has been most consistently defined according to the INTERMACS database by documentation of elevated CVP by either RHC (CVP or RA pressure >16 mmHg) or echocardiographic findings of a significantly dilated inferior vena cava with absence of inspiratory variation alongside clinical features such as jugular venous distension, peripheral oedema, presence of ascites or laboratory evidence of hepatic and/or renal dysfunction (total bilirubin >2.0 mg/dl, creatinine >2.0 mg/dl). Furthermore, RV failure is characterised as mild, moderate or severe predominantly based on signs of elevated CVP, duration of inotropic/vasodilator support, the need for temporary right-sided mechanical support device (RVAD) implantation or death from RV failure.56
Perioperative factors that influence the development of RV failure in the early post-operative period include cardioplegia during cardiopulmonary bypass, which can lead to myocardial stunning and inadequate RV unloading. Systemic inflammatory response and acute hypoxaemia during surgery can result in pulmonary vasoconstriction, PVR elevation and poor RV adaptation. While LVAD flows typically drop with acute RV failure due to reduced LV filling, filling can be maintained in some patients at the expense of high RV preload, leading to hepatic and renal congestion.
Following LVAD implantation, increased LV unloading results in increased venous return and RV preload which may increase RV wall stress. In addition, the loss of septal contribution to RV function can result in worsening RV dimensions and tricuspid regurgitation.57,58 These pathophysiological mechanisms may also contribute to the development of late RV failure.
Preoperative management for prevention of RV failure include optimisation of preload, afterload and contractility. CVP should be maintained below 15 mmHg using diuretics and inotropes such as milrinone and dopamine can be used to optimise cardiac output and improve systemic and pulmonary vascular resistance through their vasodilatory effects. Temporary mechanical support devices may be used in patients who remain haemodynamically compromised, and consideration of bridging with venoarterial extracorporeal membrane oxygenation should be assessed in patients with critical cardiogenic shock to stabilise haemodynamics and improve end-organ perfusion prior to LVAD implantation.33 When early post-operative RV failure occurs, consideration of early use of RVAD should be made to preserve LV filling and end-organ perfusion. Several studies have showed that planned or early RVAD implantation is associated with better outcomes versus delayed or rescue RVAD placement.59,60
Cardiac Tamponade
Cardiac tamponade after cardiac surgery is a medical emergency that can be reversed with accurate recognition. This complication occurs due to an abnormal accumulation of fluid in the pericardial sac, producing an increase in intrapericardial pressure that impedes normal intracardiac filling. Subsequently, the reduction of cardiac output and LVAD flows will result in a reduction of systemic perfusion, producing hypotension, tachycardia and peripheral vasoconstriction. Although clinical and echocardiographic examination are central components of diagnosis, several studies have illustrated the atypical presentation of cardiac tamponade following cardiac surgery, where conventional signs may be absent and localised collections (usually blood, serous fluid and, sometimes, infective material) may be incorrectly visualised.61 In this setting, invasive haemodynamic assessment can provide further evidence to support the diagnosis of this complication, as well excluding other causes of shock.
The elevation of intrapericardial pressure will transmit to all cardiac chambers, especially affecting the early diastolic phase and subsequently producing equalisation of all cardiac and PA diastolic pressures. An increase in RA pressure will reduce the veno-atrial gradients that determine the cardiac output. In addition, the RA is the chamber most vulnerable to compression because it has the least intracavity pressure. During inspiration, the increase in venous return and right-side filling will produce a subsequent rise in RA pressure and an opposite decrease in the left chambers filling.62,63 In the setting of low cardiac output and decreased LVAD flows, the combination of elevated right-side pressures and diastolic equalisation of RA, RV, PA and PCWP pressures should point towards the possibility of cardiac tamponade, and goal-directed bedside echocardiography should be performed. It should be noted that the classic haemodynamic findings of cardiac tamponade may not be present when the parietal pericardium is not intact; patients sometimes have localised haemorrhage/haematoma that causes right-sided chamber compression, and the haemodynamics are often atypical in that setting. Hence, a multimodal approach is necessary. Pericardiocentesis may be considered the initial therapeutic measure in this setting because repeated median sternotomy could increase the risk of mediastinitis.64
Implications of Right Heart Catheterisation in the Post-operative Period
LVADs have a positive effect in reducing PA pressures by continuously unloading the LV. ISHLT guidelines recommend serial invasive haemodynamic assessment to survey PH in patients with LVADs as a BTT strategy.65 Routine RHC is recommended 3 to 6 months after LVAD implantation to demonstrate normalisation of PA pressures and therefore allow the patient to be listed for heart transplantation. However, the ideal surveillance frequency is unknown for patients without PH prior to LVAD implantation or for those whose PA pressures and PVR have normalised post-LVAD implantation. Recent publications suggest that routine RHC may not be necessary for asymptomatic patients with normal PVR pre-LVAD implant or PVR <2.5 6 months post-LVAD implantation.66,67 Haemodynamic reassessment in LVAD patients is indicated regardless of previous measurements in the presence of new onset HF symptoms, or complications like significant aortic regurgitation or late RV failure. Non-invasive echocardiographic estimates of cardiac filling pressures in LVAD recipients have demonstrated a good correlation with right heart catheter measurements. LA volume index, mitral inflow velocities, PA systolic estimates using TR velocity, and an RA pressure estimate using the inferior vena cava can be combined in an algorithm to identify patients with elevated pulmonary capillary wedge pressures.68 However, echocardiographic assessment can be challenging due to the presence of the apically placed VAD pump, and the four measures listed above could not be obtained in about one-quarter of patients.
There is growing interest in the use of implantable haemodynamic monitoring systems, such as the CardioMEMS system (Abbott) and Titan LAP monitoring system (ISS Inc) that allow remote monitoring of HF patients.69,70 Real-time haemodynamic monitoring of LVAD recipients may allow more efficient device optimisation and detection of complications. The ongoing HEMOVAD study seeks to address its use in this setting.71
ISHLT guidelines recommend echocardiographic assessment post-LVAD implantation as an integral way to determine optimal LVAD speed, with goals including adequate LV unloading with midline LV septum and minimal mitral valve regurgitation.63 Haemodynamic assessment for LVAD speed optimisation may provide additional benefits above non-invasive evaluation alone. A combined haemodynamic and echocardiographic ramp study was described by Uriel et al.72 A baseline RHC was performed, then the device speeds were lowered to 2,300 rpm for HVAD recipients and 8,000 rpm for HM2 recipients, The speed was increased in 100 rpm and 400 rpm increments to a maximum of 3,200 rpm and 12,000 rpm, respectively. Complete pressure assessment and cardiac output via the indirect Fick method were measured at each speed, following a 2-minute period of stabilisation. Speed increases were stopped in the event of suction events or an LV end-diastolic diameter of <3 cm. The goal was to achieve PCWP <18 mmHg and CVP <12 mmHg with minimal residual mitral regurgitation and intermittent valve opening.
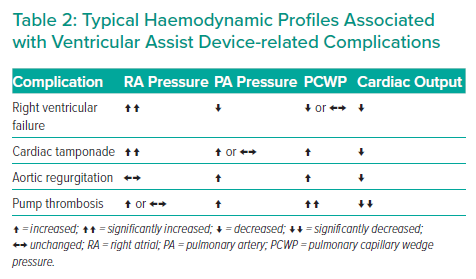
In a subsequent multicentre pilot study, the addition of serial haemodynamic ramp studies when compared to standard echocardiographic assessment alone was associated with a greater number of speed modifications and a numerical reduction in adverse event rates.73 This supports the development of a fully powered trial to assess the impact of haemodynamic optimisation on long-term patient outcomes.
Monitoring for LVAD Complications
Third generation LVADs such as the HeartMate III have demonstrated lower rates of pump thrombosis, pump failure and stroke compared to the second-generation Heartmate II device.40 However, there are other long-term complications that can lead to serious adverse outcomes and periodic RHC plays an important role in their diagnosis and risk stratification (Table 2).
Late Right Ventricular Failure
INTERMACS define late RV failure as new RV failure occurring at least 3 months post-VAD implant, characterised by evidence of raised CVP, signs and symptoms of fluid retention, such as peripheral oedema and ascites, and/or evidence of worsening hepatic or renal function. Severity is defined according to the need for readmission for intravenous diuretics and vasodilators, inotrope therapy or RVAD implant.52 Late RV failure can occur as a consequence of inadequate unloading from the LVAD due to severe aortic or mitral regurgitation, mechanical LVAD dysfunction or inadequate blood pressure management. However, in other patients late RV failure occurs in the context of normal LVAD function as a result of intrinsic RV disease.32 Regardless of the underlying cause, several studies have showed the impact of this complication as a predictor of poorer long-term outcomes during LVAD support and even after heart transplantation.74–77
Haemodynamic findings concordant with late RV failure include elevated CVP and RA pressure, equalisation of right sided pressures, low cardiac output and LVAD flows as a consequence of poor LV filling. The adequate management of this clinical scenario remains a big challenge. Pump speed optimisation by haemodynamic or echocardiographic assessment can lead to an improvement of RV function and preload optimisation using diuretics may provide a short-term amelioration of symptoms. Nevertheless, in patients with LVADs as a BTT, late RV failure may be an ominous sign that there is an urgent need for heart transplantation.
Aortic Regurgitation
Aortic regurgitation (AR) affects 25–30% of patients within the first year following LVAD implantation. Significant AR can create a continuous closed-loop circuit that leads to suboptimal LV unloading, inadequate peripheral perfusion and recurrent symptoms of HF. This phenomenon leads to an inaccurate estimation of the amount of litres per minute unloaded from the LV into the systemic circulation, producing a discrepancy between the cardiac output measured during RHC and the LVAD-derived flows. With AR progression, LV dimensions, end-diastolic pressure and mitral regurgitation will increase for a given pump speed, increasing the PCWP and PA pressures, which can subsequently lead to RV failure. In addition, AR increases aortic wall stress and has been associated with haemolysis and pump thrombosis. Nevertheless, it is unproven whether AR has an independent impact on prognosis.78,79
The main factors associated with the development and worsening of AR are a persistently closed aortic valve and prolonged duration of LVAD support. The underlying mechanisms of this alteration of the aortic root biomechanics include repetitive microtrauma of the valvular endothelium and consequently commissural fusion, tissue remodelling and ultimately valvular incompetence.80–82 Other risk factors associated with the development of AR include small body surface area, older age and being female. It is important to assess the aortic valve prior to LVAD implantation, and a concomitant procedure should be performed in the presence of at least moderate AR.83
There is no clear recommendation regarding the management of patients who develop AR under LVAD support. Targeting the lowest pump speed that allows intermittent aortic valve opening is in theory indicated to reduce AR progression, although this puts patients at risk of a low-output state and thrombotic events. In symptomatic patients, in addition to lowering LVAD pump speeds, medical therapy using vasodilators and diuretics is indicated to reduce LV afterload and aortic wall stress.79,82 Surgical correction should be considered for patients with at least moderate AR and symptoms despite OMT and device optimisation.83 Surgical alternatives include aortic valve repair, replacement or closure. Case series providing long-term outcomes data are scarce and perioperative mortality is considerable.84 In symptomatic patients with excessive surgical risk, percutaneous options may be considered, using either percutaneous occlusion devices (PODs) or transcatheter aortic valve implantation (TAVI). However, results on case series reports paint a bleak picture. In 10 patients treated with PODs, Retzer et al. reported a 70% in-hospital mortality, mainly attributable to RV failure.85 Another report on 29 LVAD patients with symptomatic AR who underwent percutaneous repair (8 TAVI and 21 PODs), showed similar benefit for AR reduction from severe to trivial, but with 31% peri-procedural mortality and an additional 25% mortality at 1 year.86
Pump Thrombosis
LVAD pump thrombosis is a life-threatening complication that requires prompt recognition and treatment. Thrombosis rates have reduced significantly with the introduction of the HM3 pump, as demonstrated in the MOMENTUM 3 trial (1% pump thrombosis rate after 24 months in the HM3 arm versus 14% in the HM2 arm.40 Clinical indicators of pump thrombosis include:
- Abrupt onset of HF symptoms, with signs of pulmonary oedema and low cardiac output.
- A sudden increase in power consumption on device interrogation.
- Signs of haemolysis – elevated lactate dehydrogenase (levels >1,000 IU/l highly suggestive of pump thrombus), bilirubinuria, elevated plasma-free haemoglobin.
- Echocardiographic signs of ineffective LV unloading – LV dilation, increased frequency of aortic valve opening, worsening mitral regurgitation or pulmonary hypertension.
- Negative ramp study using echocardiogram – increasing pump speed has no effect on LV unloading.
RHC is rarely required, but in cases where the diagnosis is less clear, for example modest power and lactate dehydrogenase rises, or in patients with poor echocardiographic windows, haemodynamic evaluation can be useful to look for evidence of the adequacy of LV unloading. LVAD flows are derived based on power consumption and speed, therefore in the presence of thrombus, an increase in pump power will result in falsely high flow estimates. Direct measurement of cardiac output via Fick or thermodilution methods will therefore provide a much more reliable estimate. Other indicators of pump thrombosis include an elevation in PA and PCW pressures. Pump exchange is usually required in confirmed pump thrombosis, but in patients who are considered too unwell for repeat surgery, treatment with heparin, glycoprotein IIb/IIIa inhibitors, direct thrombin inhibitors or thrombolytics can be considered.87,88 Continuous haemodynamic monitoring with a PA catheter to assess cardiac output and PA pressures can be useful to assess treatment response.
The Role of Right Heart Catheterisation on Myocardial Recovery Assessment
The incidence of myocardial recovery under MCS with LVADs is variable, as are the rates of relapse into HF after device explant. INTERMACS is the largest published database of durable MCS implants and reports a recovery rate of 0.9 to 1.3%.8,9 Identifying LVAD patients who experience myocardial recovery is based on assessing clinical, haemodynamic and echocardiographic parameters.
Likely indicators of LV recovery in asymptomatic LVAD patients include echocardiographic variables, such as normalisation of ejection fraction and LV dimensions as well as improvement of haemodynamic parameters that are sustained on pump speed reduction. Cardiopulmonary exercise testing is also employed as an objective measure of exercise capacity and a prognostic indicator.
RHC for recovery assessment is performed while patients are anticoagulated with warfarin and have therapeutic INR, and they are given a bolus of intravenous heparin immediately prior to reducing the VAD speed, given the increased risk of stasis and thrombus formation. The Manchester recovery protocol requires a number of criteria to be met after 15 minutes of minimal LVAD flow before considering LVAD explant (Table 3).
These variables are based on a combination of parameters from the Harefield and Berlin criteria, which represent the two European centres with the greatest experience of LVAD explant for recovery.89–91 In our centre, explant criteria were developed based on the above studies, and to date eight successful LVAD explants have been performed with no deaths or need for transplantation in the first 2 years of follow-up.89–91
Conclusion
Invasive haemodynamic assessment plays a vital role for clinicians involved in the implantation and management of patients with durable LVADs. It informs patient selection, helps to stratify perioperative risk, and can be useful in the recognition and evaluation of both short- and long-term LVAD-related complications. Larger studies are required to determine the optimal haemodynamic measures that predict risk of RV dysfunction post-LVAD implant, as well as markers of myocardial recovery that permit safe explantation of the device.